Multimodal Metabolic Imaging
Magnetic resonance spectroscopic imaging (MRSI) has been used to study biochemical processes non-invasively, although it suffers from a low sensitivity and requires long scan times. The invention of hyperpolarization (HP) techniques has shifted these limits, allowing signal enhancements of 13C-labelled metabolites by more than five orders of magnitude. However, hyperpolarized signals decay with the spin-lattice relaxation time (T1) which is on the order of a few tens of seconds, limiting the time available for signal acquisition and requiring fast signal acquisition.
Hyperpolarized [1-13C]pyruvate is most often used in vivo, because of its key role in glucose metabolism, its fast metabolic conversion to lactate, its high achievable polarization level and a relatively long T1. Tumors readily internalize and metabolize pyruvate to lactate by lactate dehydrogenase (LDH-A). The expression of LDH-A is upregulated in many tumors, which results in an increased pyruvate-to-lactate conversion that can be quantified with MRSI.
We attempt to assess the flux of glucose through the glycolytic pathway simultaneously with both FDG-PET and pyruvate imaging. In a recent study, we established and validated a workflow at a clinical whole-body PET/MR for the multiparametric characterization of the glycolytic flux in a pre-clinical MAT-B-III breast cancer model. We analyzed the in vivo correlation of FDG uptake with pyruvate-to-lactate conversion and performed a longitudinal study with a group of tumor-bearing rats to study the effect of changing tumor cellularity on metabolic PET and MRSI data (see Figure 1).
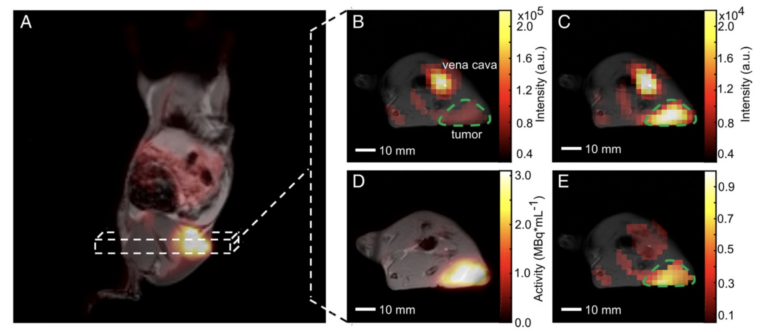
Figure 1. Qualitative comparison of simultaneously imaged FDG uptake and pyruvate-to-lactate exchange in MAT-B-III tumor-bearing rats. (A) Coronal slice of a late frame PET image overlaid on the respective slices of an MPRAGE image. Static axial images of pyruvate (B), lactate (C) and FDG (D) in the same orientation. (E) is the ratio of (C) and (B) showing a higher lactate to pyruvate ratio in the tumor than observed in the surrounding tissue, which correlates well with the high FDG uptake shown in (D). Note that for CSI images, only pixels with a signal > 20% of the maximum signal of hyperpolarized pyruvate and lactate are displayed, respectively. Note further that the pyruvate signal is about a factor of ten higher than that of lactate and that the nominal resolution of 13C-images was two-fold higher than the one of images for quantitative analysis.
In this study, single-frequency bSSFP (without multi-echoes) was used for consecutively imaging individual hyperpolarized metabolites in vitro and in vivo. The Warburg effect was imaged by observing the exchange of [1-13C]pyruvate and [1-13C]lactate. In a recent study, we investigated multi-echo-bSSFP-IDEAL at 3T. Compared to previous results, the spatial and temporal resolution was substantially improved such that observation of the metabolic conversion in one slice or 3D metabolite mapping became possible (see Figure 2).
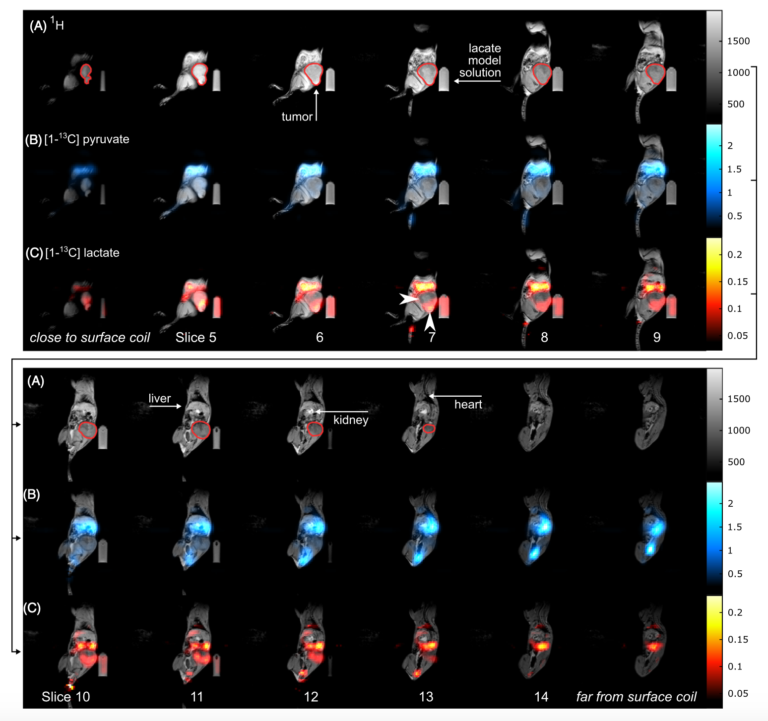
Figure 2: Sagittal slices of a 3D metabolite map. In vivo 1H MRI (A, gray) and 3D 13C metabolite maps of pyruvate (B, blue) and lactate (C, red) of a tumor bearing rat obtained with a 3D me-bSSFP. The scan was started right after injection was finished and ~45 s after dissolution. The injection lasted 26 s and the 3D scan time was 6.8 s. Strong signal of lactate and pyruvate was observed in the stomach, the blood vessels, liver, kidneys and heart (in descending order of signal intensity). The subcutaneous tumor (green outline) exhibited strong and spatially variable lactate signal, which may be a sign of metabolic heterogeneity. Note, that the image color scales start at 2.5% and 10% of the maximum intensity for pyruvate and lactate, respectively. Not all slices of the 3D volume are shown.
Further investigations of sequence variations bSSFP for fast 3D metabolite imaging are currently being investigated within the group.
Relevant publications:
- Christoph A. Müller, Christian Hundshammer, Miriam Braeuer, Jason G. Skinner, Stephan Berner, Jochen Leupold, Stephan Düwel, Stephan G. Nekolla, Sven Månsson, Adam E Hansen, Dominik von Elverfeldt, Jan H. Ardenkjaer‐Larsen, Franz Schilling, Markus Schwaiger, Jürgen Hennig, Jan‐Bernd Hövener; ‘Dynamic 2D and 3D mapping of hyperpolarized pyruvate to lactate conversion in vivo with efficient multi‐echo balanced steady‐state free precession at 3 T’, NMR in Biomedicine (2020); in press: https://doi.org/10.1002/nbm.4291
- Geoffrey J. Topping, Christian Hundshammer, Luca Nagel, Martin Grashei, Maximilian Aigner, Jason G. Skinner, Rolf F. Schulte, Franz Schilling; ‘Acquisition strategies for spatially resolved magnetic resonance detection of hyperpolarized nuclei’, Magnetic Resonance Materials in Physics, Biology and Medicine (2020); 33:221-256
- Christian Hundshammer, Miriam Braeuer, Christoph Müller, Adam Hansen, Mathias Schillmaier, Stephan Düwel, Benedikt Feuerecker, Steffen Glaser, Axel Haase, Wilko Weichert, Katja Steiger, Jorge Cabello, Franz Schilling, Jan-Bernd Hövener, Andreas Kjaer, Stephan Nekolla, Markus Schwaiger; ‘Simultaneous characterization of tumor cellularity and the Warburg effect with PET, MRI and hyperpolarized 13C-MRSI’, Theranostics (2018); 8(17):4765
Follow schillinglab on Twitter
My TweetsLatest News
-
Interview with Prof. Franz Schilling and Martin GrasheiMarch 6, 2024/0 Comments
-
Quo vadis, hyperpolarized 13C MRI?January 17, 2024/
-
New Horizons in Hyperpolarized 13C MRIJanuary 10, 2024/
-
Welcome JakobJanuary 1, 2024/
-
Happy holidaysDecember 21, 2023/
-
PHIP/dDNP Comparison on the DoverNovember 11, 2023/
-
Making the acid – base ratio visibleSeptember 4, 2023/
-
-
-
Repeatability and Reproducibility of FEXI MeasurementsJuly 12, 2023/